Welcome to our in-depth exploration of stem cell potency! In this article, we will delve into the fascinating world of stem cells, their different potency levels, and their potential applications in research and therapy. Whether you’re a scientist, a healthcare professional, or simply curious about the wonders of regenerative medicine, this article will provide valuable insights into the various types of stem cells and what sets them apart.
Key Takeaways:
- Stem cells are classified based on their potency, which refers to their ability to differentiate into different types of cells.
- The three main categories of stem cells are pluripotent, multipotent, and unipotent.
- Pluripotent stem cells have the highest differentiation potential, being capable of giving rise to almost all cell types in an organism, except for extra-embryonic cells.
- Multipotent stem cells are more restricted and can only differentiate into a limited number of cell types within a particular lineage.
- Unipotent stem cells can only differentiate into a single cell type but possess the ability to divide repeatedly.
Now, let’s take a closer look at each type of stem cell and how their potency impacts their functions and applications in the field of regenerative medicine.
Continue reading the article to gain a comprehensive understanding of stem cell potency and its implications for scientific research and therapeutic advancements.
Stay tuned for the next section where we’ll dive into the definition and characteristics of pluripotent stem cells!
Definition and Characteristics of Pluripotent Stem Cells
Pluripotent stem cells, such as embryonic stem cells (ESCs) and induced pluripotent stem cells (iPSCs), have remarkable capabilities in regenerative medicine and disease modeling. These cells possess the unique ability to differentiate into all cell types in the body, except for extra-embryonic cells.
Embryonic stem cells (ESCs) are derived from the inner cell mass of preimplantation embryos. They are pluripotent, meaning they can give rise to specialized cell types found in any organ or tissue. This pluripotent nature makes ESCs a valuable resource for research and therapeutic applications.
Induced pluripotent stem cells (iPSCs) are another type of pluripotent stem cell. Unlike ESCs, which are obtained from embryos, iPSCs are artificially reprogrammed from adult somatic cells, such as skin cells or blood cells. By introducing specific factors, these adult cells can be reprogrammed to regain pluripotency and exhibit properties similar to ESCs.
Pluripotent stem cells offer immense potential for regenerative medicine and disease modeling because they can be directed to differentiate into specific cell types for therapeutic purposes.
iPSCs, in particular, hold great promise for personalized medicine as they can be derived from a patient’s own cells, reducing the risk of immune rejection. This ability to generate patient-specific iPSCs opens new avenues for modeling diseases, screening potential drugs, and developing cell-based therapies.
Furthermore, pluripotent stem cells play a crucial role in understanding early human development and fundamental biological processes. Their extensive differentiation potential and self-renewal capabilities make them invaluable tools for uncovering the mechanisms underlying cell fate determination and tissue formation.
Features and Functions of Multipotent Stem Cells
Multipotent stem cells, such as hematopoietic stem cells, neural stem cells, and mesenchymal stem cells, play vital roles in tissue regeneration and repair. While they have a more limited differentiation potential compared to pluripotent stem cells, their unique characteristics and functions contribute to their therapeutic significance.
Hematopoietic Stem Cells
Hematopoietic stem cells (HSCs) are multipotent stem cells found in the bone marrow and umbilical cord blood. They have the ability to differentiate into various types of blood cells, including red blood cells, white blood cells, and platelets. HSCs are involved in maintaining a healthy blood system by continuously producing new blood cells throughout a person’s life.
Neural Stem Cells
Neural stem cells are multipotent stem cells found in the central nervous system. They have the remarkable ability to differentiate into neurons, the building blocks of the brain and spinal cord, as well as glial cells that provide support and protection to neurons. Neural stem cells have the potential to repair and regenerate damaged neural tissues, offering hope for treating neurodegenerative diseases and traumatic brain injuries.
Mesenchymal Stem Cells
Mesenchymal stem cells (MSCs) are multipotent stem cells present in various tissues, including bone marrow, adipose tissue, and umbilical cord. They have the capacity to differentiate into multiple cell types, such as skeletal cells (osteocytes), adipocytes (fat cells), and cartilage cells. MSCs also possess anti-inflammatory and immunomodulatory properties, making them attractive candidates for therapeutic applications in regenerative medicine, tissue engineering, and immune-related disorders.
Overall, multipotent stem cells exhibit specialized differentiation capabilities within their respective tissues. Their ability to generate a specific lineage of cell types makes them valuable resources for research, disease modeling, and potential clinical treatments.
Unipotent Stem Cells: Specialized and Restricted Differentiation
Unipotent stem cells possess a unique property of being able to differentiate into a single cell type. Although their differentiation capabilities are limited compared to pluripotent and multipotent stem cells, they play a crucial role in specific tissues and organs. The two prominent examples of unipotent stem cells are skin stem cells and muscle stem cells.
Skin Stem Cells: Formation of the Protective Layer
Skin stem cells, also known as epidermal stem cells, are unipotent cells found in the outermost layer of the skin. These cells are responsible for maintaining and regenerating the protective layer of the skin, known as the epidermis. Skin stem cells produce keratinocytes, which are the main cells that form the epidermis.
These unipotent stem cells ensure the continuous renewal and repair of the skin throughout a person’s lifetime. They are crucial for wound healing, as they rapidly proliferate and differentiate into keratinocytes to replace damaged or lost skin cells.
Muscle Stem Cells: Generation of New Muscle Cells
Muscle stem cells, also referred to as satellite cells, are unipotent cells that reside in the skeletal muscles. These unique cells play a vital role in the regeneration and repair of muscle tissues. When muscles are injured or experience damage, muscle stem cells divide and differentiate into new muscle cells, aiding in the healing process.
By replenishing damaged or lost muscle cells, unipotent muscle stem cells contribute to the restoration of muscle function and overall tissue integrity. They are essential for maintaining muscle strength, supporting physical activity, and recovering from injuries.
Unipotent stem cells, although limited in their differentiation potential, offer significant potential for regenerative medicine applications. Their ability to divide repeatedly and generate specialized cell types makes them valuable in treating specific tissues and organs.
Stem Cell Potency: A Developmental Hierarchy
In the realm of stem cells, potency is not a one-size-fits-all concept. Stem cells follow a developmental hierarchy, where their potential to differentiate into various cell types changes as they progress along the developmental pathway. This hierarchy encompasses totipotent stem cells, pluripotent stem cells, and lineage-specific multipotent stem cells.
At the pinnacle of this hierarchy are totipotent stem cells, which possess the remarkable ability to give rise to all cell types found in an organism, including extra-embryonic cells. These cells are only present in the earliest stages of development, such as the fertilized egg and the subsequent divisions that form the blastocyst. Their unique potency enables the formation of an entire organism.
Next in line are pluripotent stem cells, which retain the capability to differentiate into any cell type in the body, with the exception of extra-embryonic cells. Pluripotent stem cells have garnered significant attention in the field of regenerative medicine due to their potential to generate specialized cells for therapeutic purposes. Two prominent examples of pluripotent stem cells are embryonic stem cells (ESCs) derived from the inner cell mass of preimplantation embryos, and induced pluripotent stem cells (iPSCs) that are artificially reprogrammed from adult somatic cells.
Further down the developmental hierarchy are lineage-specific multipotent stem cells, which have a more limited differentiation potential compared to pluripotent stem cells. These stem cells can differentiate into a specific range of cell types within a particular lineage, such as blood cells, neurons, or skeletal cells. The multipotency of these cells enables tissue-specific regeneration and repair.
The importance of understanding this developmental hierarchy lies in comprehending the changing capabilities of stem cells as they progress towards becoming mature, differentiated cells. This hierarchy serves as a blueprint for developmental potential and guides the utilization of different stem cell types in various research and therapeutic applications.
Differentiation Potential of Stem Cell Types
Stem Cell Type | Differentiation Potential |
---|---|
Totipotent Stem Cells | Can differentiate into all cell types, including extra-embryonic cells |
Pluripotent Stem Cells | Can differentiate into all cell types except extra-embryonic cells |
Lineage-Specific Multipotent Stem Cells | Can differentiate into a limited range of cell types within a specific lineage |
Illustration of Stem Cell Potency Hierarchy
“The developmental hierarchy of stem cell potency provides insights into the capabilities of different stem cell types and their potential applications in research and medicine.”
Understanding the developmental hierarchy of stem cells unlocks the potential for harnessing their regenerative capacities in a myriad of endeavors. It paves the way for advancements in stem cell therapy, regenerative medicine, and tissue engineering. As researchers delve deeper into this hierarchy, new discoveries will continue to expand our knowledge and shape the future of stem cell-based therapeutics.
Reprogramming and Induction of Pluripotency
The field of stem cell research has witnessed a major breakthrough with the discovery of nuclear reprogramming methods. Two of the most significant techniques are somatic cell nuclear transfer and the induction of pluripotency through the expression of specific transcription factors.
Nuclear reprogramming involves resetting the epigenetic marks on a cell’s DNA, allowing it to regain the developmental potential it had lost during differentiation. Through this process, somatic cells can be reprogrammed to achieve a totipotent or pluripotent state.
Somatic cell nuclear transfer (SCNT) is a technique that involves the transfer of the nucleus of a mature somatic cell into an enucleated egg cell. The resulting reconstructed egg cell possesses the totipotent potential to give rise to all cell types in an organism. This method was first successfully demonstrated with the birth of Dolly the sheep in 1996, and it has since played a crucial role in stem cell research.
Induced pluripotent stem cells (iPSCs) represent a significant breakthrough in the field. iPSCs are generated by reprogramming adult somatic cells through the introduction of specific transcription factors. These factors, such as OCT4, SOX2, KLF4, and c-MYC, can reset the gene expression patterns of adult cells, reverting them to a pluripotent state that closely resembles embryonic stem cells.
The use of iPSCs is particularly promising as they can be derived from a patient’s own cells, eliminating concerns associated with immune rejection. This makes iPSCs valuable for disease modeling, drug discovery, and regenerative medicine.
Reprogramming techniques have opened up new avenues for studying and harnessing the power of stem cells, providing researchers with a renewable and ethical source of pluripotent cells for various applications.
Quotes:
“Nuclear reprogramming techniques have revolutionized the field of stem cell research, offering new possibilities in disease modeling and regenerative medicine.” – Dr. Jane Anderson, Stem Cell Researcher
Key Points:
- Nuclear reprogramming enables the reprogramming of somatic cells to achieve a totipotent or pluripotent state.
- Somatic cell nuclear transfer (SCNT) involves transferring the nucleus of a mature somatic cell into an enucleated egg cell.
- Induced pluripotent stem cells (iPSCs) are generated by reprogramming adult somatic cells through the expression of specific transcription factors.
- iPSCs have great potential for disease modeling, drug discovery, and regenerative medicine.
- Reprogramming techniques have opened up new avenues for studying and harnessing the power of stem cells.
Functional Assays for Evaluating Stem Cell Potency
Evaluating the potency of stem cells requires the use of functional assays and the identification of molecular markers. Functional assays are essential tools that assess the ability of stem cells to differentiate into specific cell types and evaluate their developmental states and functional capacities. These assays provide valuable insights into the potential of stem cells for various applications, such as regenerative medicine and disease modeling.
Molecular markers play a crucial role in defining and characterizing the pluripotency states of different types of stem cells. They include transcription factors, microRNAs, and epigenetic chromosomal modifiers, which are specific molecules that indicate the differentiation potential and lineage commitment of stem cells. By identifying and analyzing these molecular markers, researchers can better understand the pluripotency states of stem cells and harness their therapeutic potential.
To illustrate the importance of functional assays and molecular markers in evaluating stem cell potency, consider the following table:
Assay Type | Advantages | Limitations |
---|---|---|
Teratoma Formation Assay | Provides in vivo evidence of pluripotency | Requires an animal model and lengthy observation period |
Embryoid Body Formation Assay | Allows for the assessment of multiple germ layer differentiation | Requires complex culture conditions and lacks specificity |
Gene Expression Profiling | Provides quantitative data on pluripotency markers | Does not capture dynamic changes in pluripotency |
Functional Single-Cell Assays | Enables the evaluation of heterogeneity within stem cell populations | May require specialized equipment and expertise |
Different Potency States of Pluripotent Stem Cells
Pluripotent stem cells, the versatile building blocks of life, exist in various potency states that determine their potential to differentiate into specific cell lineages. These distinct states, namely the ground state, naive state, and primed state, encompass different molecular and functional characteristics that researchers are actively exploring and characterizing in their quest to unlock the full potential of pluripotent stem cells.
In the ground state, pluripotent stem cells exhibit a pristine and undifferentiated state, poised for development. They retain the ability to differentiate into specific cell types, but are not committed to any particular lineage.
The naive state represents an earlier stage of pluripotency, characterized by greater developmental plasticity. Stem cells in this state possess the potential to differentiate into a wider range of cell types and are more reminiscent of the early stages of embryonic development.
On the other end of the spectrum, stem cells in the primed state have undergone some level of lineage specification. They are more restricted in their differentiation potential and display a preference for specific cell lineages. These cells are often associated with later stages of embryonic development.
The identification and understanding of these different potency states of pluripotent stem cells hold immense significance in both clinical and research applications. By deciphering the molecular markers and transcription factors associated with each state, researchers can effectively harness the unique properties of pluripotent stem cells for various therapeutic and regenerative medicine purposes.
For instance, the transcription factors OCT4, SOX4, and NANOG have been identified as potential markers to define and characterize the different potency states of pluripotent stem cells. These markers allow scientists to distinguish between ground state, naive state, and primed state pluripotent stem cells, enabling more targeted approaches in their utilization.
Research into the different potency states of pluripotent stem cells not only provides valuable insights into the fundamental biology of these remarkable cells but also paves the way for the development of innovative strategies in regenerative medicine and disease modeling.
Potency State | Description |
---|---|
Ground State | Undifferentiated state with the ability to differentiate into specific cell types |
Naive State | Greater developmental plasticity and potential to differentiate into a wide range of cell types |
Primed State | Some level of lineage specification with a preference for specific cell lineages |
The image above visually represents the different potency states of pluripotent stem cells, emphasizing their versatility and potential for differentiation into various cell lineages.
Functional Assays for Pluripotency Evaluation
When it comes to assessing the pluripotency of stem cells, functional assays play a critical role. These assays help researchers understand the differentiation potential and developmental capabilities of pluripotent stem cells. Two commonly used functional assays for evaluating pluripotency are the teratoma formation assay and in silico analysis.
The Teratoma Formation Assay
The teratoma formation assay is a widely recognized method for assessing the differentiation potential of pluripotent stem cells. In this assay, pluripotent stem cells are injected into immune-compromised animals, such as mice, to form tumors called teratomas. These teratomas contain a complex mix of tissues derived from the pluripotent stem cells, including cell types representing the three primary germ layers – ectoderm, endoderm, and mesoderm (Aghabozorgi et al., 2020). By analyzing the composition and diversity of tissues within the teratomas, researchers can determine the pluripotent nature of the stem cell population (Image 9).
In Silico Analysis
In recent years, in silico analysis has emerged as an alternative approach to assess pluripotency and differentiation potential. This method involves computational analysis and modeling of stem cell characteristics and behavior. By utilizing bioinformatics and statistical algorithms, researchers can analyze large-scale gene expression data to identify specific genetic signatures associated with pluripotency and differentiation (Otiniano et al., 2016). This computational approach provides valuable insights into the molecular mechanisms underlying pluripotency and aids in the prediction of cell fate decisions.
In silico analysis offers a cost-effective and time-efficient method for evaluating pluripotency, complementing traditional experimental approaches (Otiniano et al., 2016).
Advancing Diagnostic Tools and Standardized Assays
As the field of stem cell research continues to evolve, the development of more concrete definitions, diagnostic tools, and standardized assays is crucial. It is essential to establish clear criteria and guidelines that enable the reliable assessment of pluripotency in different stem cell populations. This includes the identification of specific molecular markers, functional assays, and bioinformatics tools that can ensure accurate characterization and categorization of pluripotent stem cells.
Click here for references
Assay | Description |
---|---|
Teratoma Formation Assay | An experimental method involving the injection of pluripotent stem cells into immune-compromised animals to form teratomas, which contain cell types representing the three primary germ layers – ectoderm, endoderm, and mesoderm. |
In Silico Analysis | A computational approach that uses bioinformatics and statistical algorithms to analyze large-scale gene expression data, enabling the identification of genetic signatures associated with pluripotency and differentiation potential. |
Stem Cell Potency in Different Species
The understanding of stem cell potency extends across different species, including mice, humans, and other mammals. Studies conducted in mice and human stem cells have provided valuable insights into the functional assays and markers used to define and assess stem cell potency.
While there may be variations in the specific assays and markers used, the fundamental principles of stem cell potency remain consistent across species. Ongoing research in various mammalian systems continues to contribute to our understanding of stem cell biology and their potential applications.
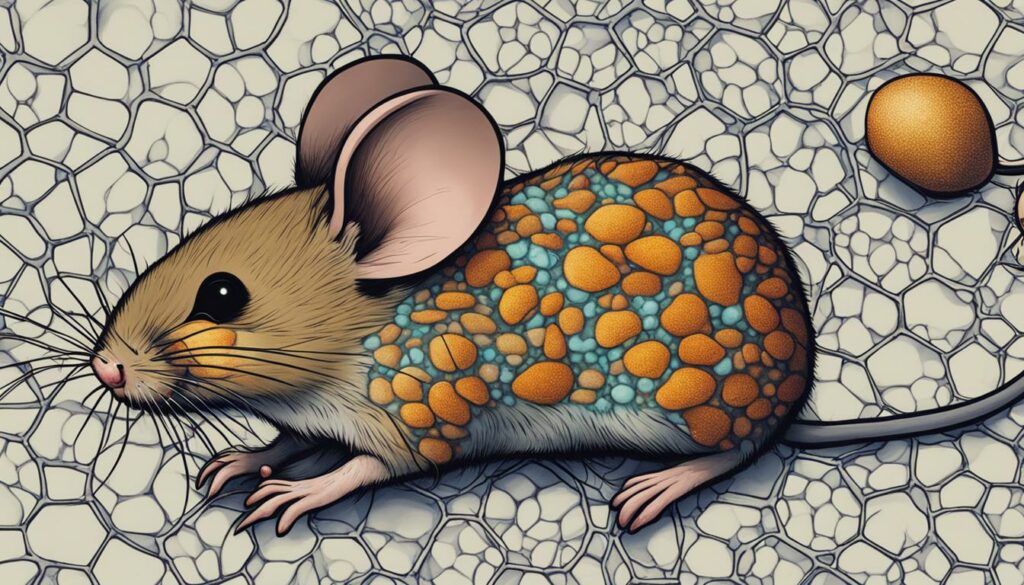
Conclusion
Understanding the distinctions between totipotent, pluripotent, and multipotent stem cells is crucial for advancing scientific research, regenerative medicine, and therapeutic applications. Each type of stem cell offers unique capabilities and limitations in terms of differentiation potential and tissue-specific applications.
Stem cell therapy holds great promise for treating various diseases and injuries by harnessing the regenerative potential of different stem cell types. The developmental potential of these remarkable cells provides a powerful tool for repairing and regenerating damaged tissues, rejuvenating organs, and restoring bodily functions.
As researchers continue to explore the therapeutic applications of stem cell therapy, ongoing development and optimization of techniques and procedures will enhance the efficacy and safety of this innovative treatment approach. The potential for stem cell therapy to revolutionize regenerative medicine and provide targeted treatments for a wide range of conditions is a testament to the remarkable potential of these cells.
The future of stem cell therapy lies in unlocking their full developmental potential and understanding the intricacies of their differentiation pathways. With continued research and advancements in the field, we can expect to see further breakthroughs in stem cell-based therapies, bringing new hope to patients and paving the way for transformative medical interventions.
FAQ
What are stem cells?
Stem cells are undifferentiated cells that have the ability to differentiate into different types of cells in the body.
What is the difference between pluripotent and multipotent stem cells?
Pluripotent stem cells can differentiate into all cell types in the body, while multipotent stem cells can only differentiate into a limited number of cell types within a specific lineage.
What are pluripotent stem cells?
Pluripotent stem cells, such as embryonic stem cells (ESCs) and induced pluripotent stem cells (iPSCs), have the ability to differentiate into all cell types in the body (except for extra-embryonic cells).
What are multipotent stem cells?
Multipotent stem cells can differentiate into a specific lineage of cell types within a particular tissue, such as hematopoietic stem cells, neural stem cells, and mesenchymal stem cells.
What are unipotent stem cells?
Unipotent stem cells can only differentiate into a single cell type, such as skin stem cells or muscle stem cells.
What is the hierarchy of stem cell potency?
Stem cell potency follows a developmental hierarchy, starting with totipotent stem cells that can give rise to all cell types, followed by pluripotent stem cells and then lineage-specific multipotent stem cells.
How are pluripotent stem cells generated?
Pluripotent stem cells can be derived from the inner cell mass of preimplantation embryos (ESCs) or artificially reprogrammed from adult somatic cells (iPSCs).
How are stem cells’ potency evaluated?
Stem cell potency is evaluated through functional assays, such as teratoma formation assay, and the identification of molecular markers that define their pluripotency states.
What are the different potency states of pluripotent stem cells?
Pluripotent stem cells can exist in ground state, naive state, or primed state, each characterized by specific molecular and functional characteristics.
How can pluripotency be assessed in stem cells?
Pluripotency can be assessed through functional assays like the teratoma formation assay and in silico analysis, along with the identification of molecular markers.
Are stem cell potency characteristics consistent across different species?
While there may be variations in specific assays and markers used, the fundamental principles of stem cell potency remain consistent across species, including mice, humans, and other mammals.
What are the potential applications of stem cell therapy?
Stem cell therapy holds great promise for regenerative medicine and the treatment of various diseases and injuries by harnessing the regenerative potential of different types of stem cells.